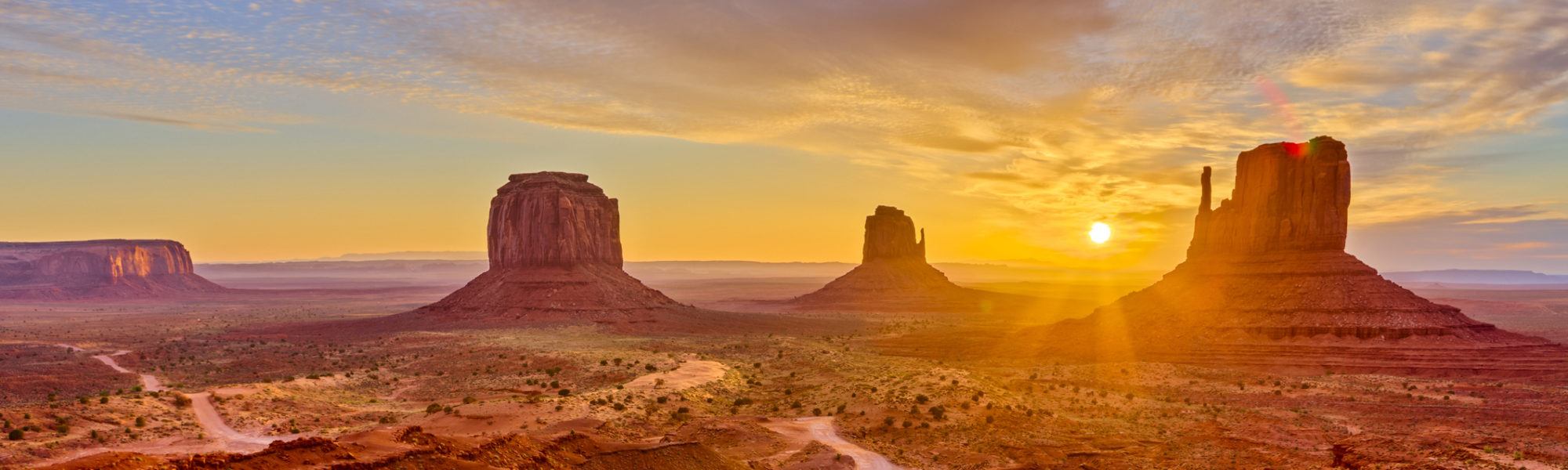
Assessment of Cerebral Blood Flow with CT Perfusion and PET Before and After STA-MCA Bypass for Symptomatic Carotid Occlusion: Case Report
John E. Wanebo, MD*
Joseph M. Zabramski, MD
Giuseppe Lanzino, MD**
Shahram Partovi, MD+
Robert F. Spetzler, MD
Divisions of Neurological Surgery and +Neuroradiology, Barrow Neurological Institute, St. Joseph’s Hospital and Medical Center, Phoenix, Arizona
**Department of Neurosurgery, University of Illinois College of Medicine at Peoria, Peoria, Illinois
Current Address:
*Naval Medical Center, San Diego, California
This study compared the use of PET and CT perfusion imaging before and after cerebral revascularization. PET and CT perfusion images were obtained in a patient with unilateral carotid occlusion before and after STA-MCA bypass was performed. Improved cerebral blood flood and reduced mean transit times were noted on the side of the STA-MCA bypass. These results correlated with the PET and CT perfusion images. This case demonstrates the possibility of using CT perfusion to assess cerebral blood flow pre- and postoperatively in patients undergoing cerebral revascularization for carotid occlusion.
Key Words: cerebral blood flow, computed tomography perfusion, positron emission tomography, revascularization
Abbreviations Used: ACA, anterior cerebral artery; CBF, cerebral blood flow; COSS, Carotid Occlusion Surgery Study; CT, computed tomography; EC-IC, extracranial-intracranial; ICA, internal carotid artery; MCA, middle cerebral artery; MR, magnetic resonance; PET, positron emission tomography; STA, superficial temporal artery
Evaluating the need for and success of revascularization is evolving.[6] PET, single photon emission CT, and xenon CT have been standard techniques used to assess cerebral hemodynamics and stroke risk.[6,8,10,13,18-20,22,23,25,26] However, these imaging techniques are unavailable at most centers. Advances in CT scanners and software have facilitated the development of CT perfusion techniques, which provide a CBF study using a standard spiral CT scanner.[14]
Qualitative brain perfusion imaging is based on two methods: the diffusible tracer model such as in PET or the tracer kinetic model such as in CT perfusion. Until recently, the only quantitative method for assessing cerebral perfusion has been PET, which has served as the gold standard in this field. Validation models have shown that techniques such as CT perfusion may also help to assess brain perfusion quantitatively.[21] CT perfusion is a relatively new modality that permits the microcirculation of the brain to be evaluated without the need for expensive radiotracers or intra-arterial access as needed in PET. It is performed on standard spiral (or better) CT scanners using an intravenous bolus injection of iodinated contrast followed by dynamic scanning.
We describe a patient who underwent cerebral revascularization after a MCA stroke caused by unilateral carotid occlusion who was evaluated by both PET and CT perfusion scanning before and after surgery. The information about CBF provided by CT perfusion was comparable to that obtained with PET. CT perfusion imaging may be an alternate modality for evaluating compromised CBF.
Illustrative Case
A 66-year-old, right-handed, white man had sustained a cardioembolic stroke in the right MCA 15 months before his admission following a myocardial infarction a month earlier. Initially, the patient was hemiplegic on the left side and had partial left homonymous hemianopsia. He had improved to the point of ambulation with a cane. His medical history was significant for hypertension, hypercholesterolemia, and a seizure disorder.
Physical examination revealed a thin male in no apparent distress. His general physical examination was unremarkable. He was alert and oriented times three with clear, fluent speech. His cranial nerves were intact except for trace central left facial palsy, and his tongue protruded to the left. He had left temporal quadrantanopsia. On motor examination his strength was 4/5 on the left. His deep tendon reflexes were 3+ on the left side and 2+ on the right. He had a positive Babinski sign on plantar stimulation of the left foot.
He underwent CT perfusion imaging. To obtain CT perfusion images, dynamic scanning was performed at a slice location where the territories of the ACA, MCA, and posterior cerebral artery could be identified. This level typically includes the basal ganglia. During a bolus intravenous injection of iodinated contrast (40 ml), axial images were rapidly obtained at the same slice location. With mulitdetector technology, more than one slice location was imaged even though the scanner table is stationary. The resultant images were postprocessed on a workstation to yield CT perfusion images. The entire process typically required less than 15 minutes. The PET studies were performed with an oxygen tracer [15O]. Scan times were 1 minute.
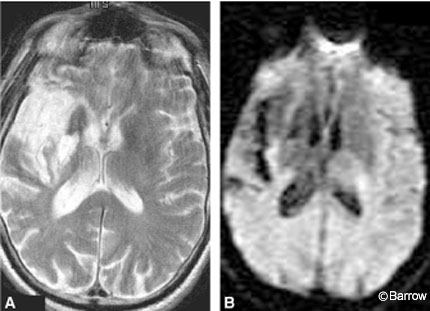
Preoperative MR imaging of the brain showed a chronic infarct in the distribution of the right MCA (Fig. 1). MR angiography of the neck and brain suggested complete occlusion of the right ICA. Preoperative digital subtraction angiography confirmed the right ICA occlusion and further delineated filling of the right ACA from the left via the anterior communicating artery (Fig. 2). Trace retrograde filling of the right MCA via collaterals from the right ophthalmic artery was also noted.
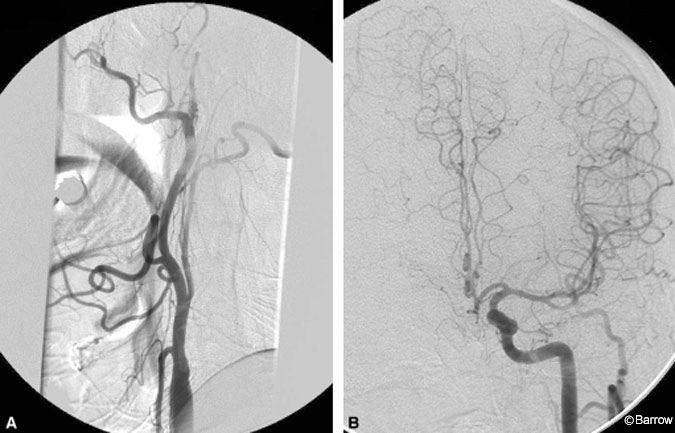
Preoperative CT perfusion imaging demonstrated hypoperfusion and prolonged transit time within the right hemisphere in a region that extended beyond the distribution of the right MCA stroke as visualized on diffusionweighted MR imaging (Fig. 3A and B). Preoperative PET also revealed right hemisphere hypoperfusion in a distribution comparable to that of the CT perfusion study (Fig. 3C).
The patient underwent a right STAMCA bypass procedure uneventfully. His postoperative examination was unchanged from his preoperative status. At a 2-month follow-up examination, the patient reported that his left hand function had improved and that he was less fatigued than before surgery.
Compared to the patient’s preoperative study, postoperative CT perfusion showed dramatic improvement in CBF and mean transit time within the right hemisphere (Fig. 4A and B). Similarly, postoperative PET demonstrated significant improvement in CBF in the right hemisphere comparable to the results obtained on CT perfusion imaging (Fig. 4C).
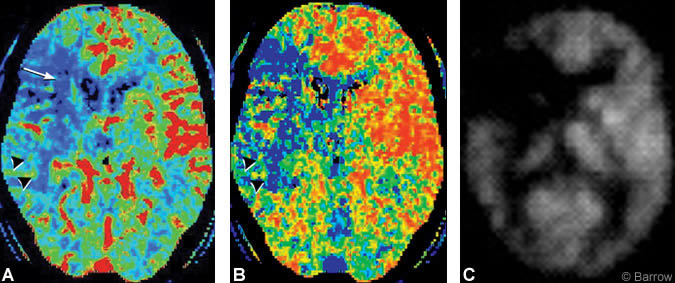
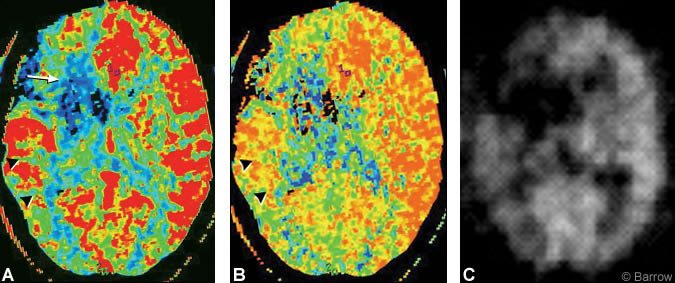
Discussion
After its introduction for clinical use in the late 1960s, EC-IC bypass was commonly applied to patients with carotid occlusion in an attempt to prevent subsequent stroke.[3,24] However, a multicenter randomized trial of surgery compared to medical management failed to demonstrate that STA-MCA bypass surgery prevented stroke.[17] The EC-IC bypass trial was criticized for failure to evaluate the subgroup of patients with reduced cerebral perfusion whom might have benefited from cerebral revascularization.[2,16,26]
The St. Louis Carotid Occlusion Study identified a subgroup of symptomatic patients with carotid occlusion who had impaired cerebral perfusion as measured by increased oxygen-extraction fraction (stage 2 hemodynamic failure) on PET.[6] A new EC-IC bypass study
(Carotid Occlusion Surgery Study, COSS) has been funded by the National Institutes of Health and is presently enrolling patients at 30 centers across the United States. Should revascularization prove effective in preventing stroke in this population, evaluation of CBF will become routine for patients with carotid occlusion. Because the 15O isotope used for PET imaging of CBF and measurement of oxygen-extraction fraction has a half-life of only 2 minutes, it requires on-site production and is available at only a limited number of centers. CT perfusion may offer a more readily available method of evaluating cerebral hemodynamics.
In this case report, CBF was evaluated with PET using water labeled with 15O and compared to CBF as measured by CT perfusion. However, CT perfusion imaging was not compared to the oxygen extraction fraction. The latter was the gold standard for evaluating type 2 hemodynamic failure in the St. Louis Carotid Occlusion Study. Whether the changes in blood flow seen with CT perfusion would correlate with changes in the oxygen extraction fraction with PET remains to be tested.
This is a report of the utility of CT perfusion in its current state as a tool to assess CBF after STA-MCA bypass grafting in a patient with symptomatic carotid occlusion. Studies with PET have shown postoperative improvement of cerebral hemodynamics after STAMCA bypass.[1,4,5,9,11,12,15] This case demonstrates the possibility of using CT perfusion to assess CBF before and after surgery in patients undergoing revascularization for carotid occlusion. In this case the patient improved clinically after bypass, nicely correlating with the improved blood flow seen on his CT perfusion images.
Further study of PET and CT perfusion imaging in a prospective, controlled fashion to determine the true comparability of the two modalities for analysis of CBF is necessary. Although not performed in the patient reported here, the effect of an acetazolamide challenge on CBF can be measured with CT perfusion imaging and may be an appropriate comparison with PET studies evaluating the oxygen extraction fraction. Carotid occlusion patients may provide a substrate for assessing the utility of CT perfusion imaging in comparison with other modalities such as PET. CT perfusion also may be used to evaluate CBF in other candidates for revascularization, such as those with moyamoya disease or those with complex aneurysms or tumors who undergo balloon test occlusion to evaluate the feasibility of vessel sacrifice. CT perfusion may be a test more readily implemented in hospitals than xenon CT or PET using existing CT scanners with the appropriate software.
References
- Baron JC, Bousser MG, Rey A, et al: Reversal of focal “misery-perfusion syndrome” by extra-intracranial arterial bypass in hemodynamic cerebral ischemia. A case study with 15O positron emission tomography. Stroke 12:454-459, 1981
- Day AL, Rhoton AL Jr, Little JR: The extracranialintracranial bypass study. Surg Neurol 26:222-226, 1986
- Donaghy RM: Neurologic surgery. Surg Gynecol Obstet 134:269-270, 1972
- Gibbs JM, Wise RJ, Thomas DJ, et al: Cerebral haemodynamic changes after extracranial-intracranial bypass surgery. J Neurosurg Psychiatry 50:140-150, 1987
- Grubb RL Jr: Management of the patient with carotid occlusion and a single ischemic event. Clin Neurosurg 33:251-280, 1986
- Grubb RL Jr, Derdeyn CP, Fritsch SM, et al: Importance of hemodynamic factors in the prognosis of symptomatic carotid occlusion. JAMA 280:1055-1060, 1998
- Grubb RL Jr, Powers WJ: Risks of stroke and current indications for cerebral revascularization in patients with carotid occlusion. Neurosurg Clin North Am 36:473-487, 2001
- Hasegawa Y, Yamaguchi T, Tsuchiya T, et al: Sequential change of hemodynamic reserve in patients with major cerebral artery occlusion or severe stenosis. Neuroradiology 34:15-21, 1992
- Kawamura S, Sayama I, Yasui N, et al: Haemodynamic and metabolic changes following extraintracranial bypass surgery. Acta Neurochir (Wien) 126:135-139, 1994
- Kleiser B, Widder B: Course of carotid artery occlusions with impaired cerebrovascular reactivity. Stroke 23:171-174, 1992
- Leblanc R, Tyler JL, Mohr G, et al: Hemodynamic and metabolic effects of cerebral revascularization. J Neurosurg 66:529-535, 1987
- Powers WJ, Martin WR, Herscovitch P, et al: Extracranial-intracranial bypass surgery: hemodynamic and metabolic effects. Neurology 34:1168-1174, 1984
- Powers WJ, Tempel LW, Grubb RL Jr: Influence of cerebral hemodynamics on stroke risk: one-year follow-up of 30 medically treated patients. Ann Neurol 25:325-230, 1989
- Rother J, Jonetz-Mentzel L, Fiala A, et al: Hemodynamic assessment of acute stroke using dynamic single-slice computed tomographic perfusion imaging. Arch Neurol 57:1161-1166, 2000
- Samson Y, Baron JC, Bousser MG, et al: Effects of extra-intracranial arterial bypass on cerebral blood flow and oxygen metabolism in humans. Stroke 16:609-616, 1985
- Schmiedek P, Piepgras A, Leinsinger G, et al: Improvement of cerebrovascular reserve capacity by EC-IC arterial bypass surgery in patients with ICA occlusion and hemodynamic cerebral ischemia. J Neurosurg 81:236-244, 1994
- The EC/IC Bypass Study Group: Failure of extracranial-intracranial arterial bypass to reduce the risk of ischemic stroke. Results of an international randomized trial. N Engl J Med 313:1191-1200, 1985
- Vernieri F: Outcome of carotid artery occlusion is predicted by cerebrovascular reactivity. Stroke 30:593-598, 1999
- Webster MW, Makaroun MS, Steed DL, et al: Compromised cerebral blood flow reactivity is a predictor of stroke in patients with symptomatic carotid artery occlusive disease. J Vasc Surg 21:338-344, 1995
- Widder B, Kleiser B, Krapf H: Course of cerebrovascular reactivity in patients with carotid artery occlusions. Stroke 25:1963-1967, 1994
- Wintermark M, Thiran JP, Maeder P, et al: Simultaneous measurement of regional cerebral blood flow by perfusion CT and stable xenon CT: A validation study. Am J Neuroradiol 22:905-914, 2001
- Yamauchi H, Fukuyama H, Nagahama Y, et al: Evidence of misery perfusion and risk for recurrent stroke in major cerebral arterial occlusive diseases from PET. J Neurol Neurosurg Psychiatry 61:18-25, 1996
- Yamauchi H, Fukuyama H, Nagahama Y, et al: Significance of increased oxygen extraction fraction in five-year prognosis of major cerebral arterial occlusive diseases. J Nucl Med 40:1992-1998, 1999
- Yasargil MG: Microsurgery Applied to Neurosurgery. New York: Academic Press, 1969
- Yokota C, Hasegawa Y, Minematsu K, et al: Effect of acetazolamide reactivity on [corrected] long-term outcome in patients with major cerebral artery occlusive diseases. Stroke 29:640-644, 1998
- Yonas H, Smith HA, Durham SR, et al: Increased stroke risk predicted by compromised cerebral blood flow reactivity. J Neurosurg 79:483-489, 1993